Hybrid Solar Energy Systems
Commercial Systems
Semi-Transparent Luminescent BIPV Windows
By James Walshe and Philippe Lemarchand, Technological University Dublin, Ireland
Product Description
Brief Concept Description
The integration of photovoltaics into buildings facades offers the growing possibility of making the structures in our society play a more active role in our transition towards a sustainable economy. Luminescent building integrated photovoltaic windows (L-BIPV) are semi-transparent systems based on luminescent down-shifting species (LDS) which are combined with photovoltaic solar cells. LDS materials absorb diffused and direct light within the ultraviolet region (200nm– 400nm) and re-radiate it to a tuned wavelength band within the 500nm-700nm range. The capability to tune the optical characteristics of the luminescent species considered to a specific region of the spectrum (650nm -700nm) where the PV cells responsivity is higher and the human eyes responsivity is low, is key for the semitransparent hybrid device to provide some of the indoor lighting requirements and enhance the energy conversion from the photoluminescence.
Two different designs of L-BIPV were developed (Figure 70): in LDS-PV systems, a semi-transparent PV cell is combined directly underneath the LDS layer. In luminescent solar concentrator (LSC) systems, instead, light is captured and re-emitted by the LDS species and concentrated by total internal reflection to small and highly efficient PV cells located within a window edge cell. In comparison to the use of large semi-transparent photovoltaic cells integrated in the glazing, the use of thin strip silicon within the window edges offers advantages in terms of costs, glazing transmission as well as overall light to power conversion efficiency of the system.
L-BIPV systems can potentially replace or be added to conventional window to produce electricity, thus reducing the building energy consumption and carbon emission, improve indoor light and thermal comfort and provide colourful architectural designs.
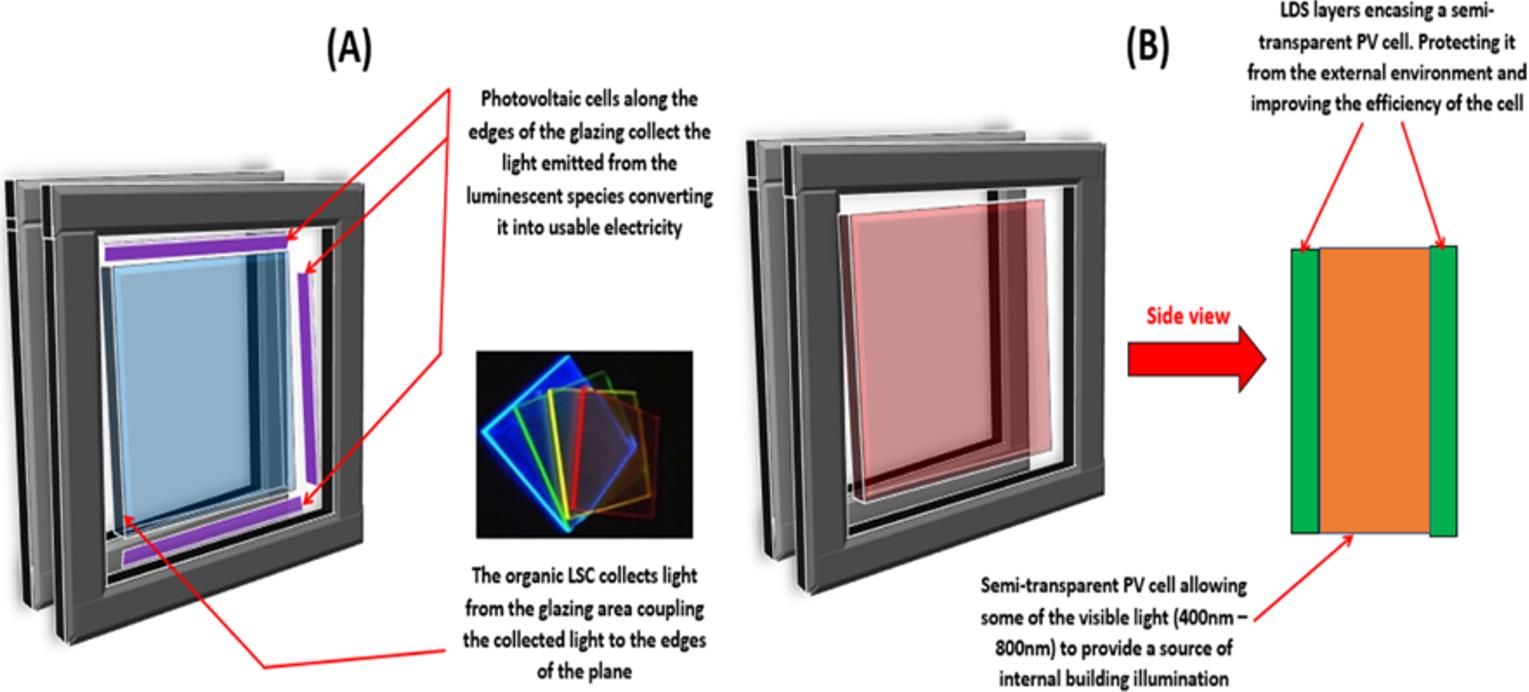
Figure 70. Two different luminescent building-integrated photovoltaic windows design options (A) building integrated LSC window and (B) building integrated LDS-PV window.
Interest in semi-transparent PV devices has undergone a massive revival in the last 20 years due to the ability of such devices to be fully integrated into building elements such as skylights, facades, windows and even walls (Saifullah, Gwak, and Yun 2016). Semi-transparent PV cell devices based on perovskite, thin-film and organic technologies are on the verge of becoming commercial available (Heim, Knera, and Machniewicz 2015; RomeroGómez et al. 2015). Current approaches have been limited due to issues inherent in each technology, in terms of its scalability and long-term stability when exposed to in-situ environmental conditions (Bouvard, Vanzo, and Schüler 2015; Klampaftis et al. 2009). Currently, no window design incorporating LDS features is commercially available, with such structures presently confined to a research and development state. Efforts have been consolidated into a multi-teared design approach, attempting to reduce the losses associated with each design element while harnessing a synergistic interaction between components within the structure to deliver a commercially viable L-BIPV design.
Architectural and Technological Integration into the Envelope
Architectural and technological integration into the envelope L-BIPV windows can be integrated into building envelopes similarly to traditional BIPV systems. LDS and similar spectral conversion technologies including LSC underwent a revival in the last decade with the development of new design strategies. Exploiting the energy transfer between nanostructures and luminescent species, engineering the molecules around the device and incorporating multiple chemical species are just a few of the routes being explored to deliver a commercially viable solution to the limited spectral bandwidth problem facing PV technology.
LSC systems offer a greater enhancement in the performance of BIPV as compared to their more readily incorporable LDS-PV counterparts. The lack of scalability and poor structure stability currently limit such devices from being commercially available. However, industries such as Philips are working on commercializing a building integrated version of the technology through funding initiatives aimed at pioneering researchers within the area.
LDS-PV designs offer a lower level of enhancement, but they are more readily scalable and have yet to encounter the size limitations imposed by concentrating designs explored so far. The addition of an LDS layer directly onto a cell creates a series of additional loss mechanisms which need to be overcome for the structure to offer an enhancement to the underlying PV cell. The poor optical properties and non-ideal photoluminescent materials available represent the primary introduced additional losses.
In terms of architecture, L-BIPV devices are readily adoptable into existing building plans. Similar transparent devices are already in operation within the illustrious skyline of Hong Kong. To maximise the synergy between the electricity generated by the devices and the interior illumination provided, the trade-off between the two warrants optimization. Parameters to consider include i) outdoor environmental conditions ii) building orientation, iii) window dimensions, iv) window transparency of the choice of solar cell and v) aesthetic and indoor lighting requirements.
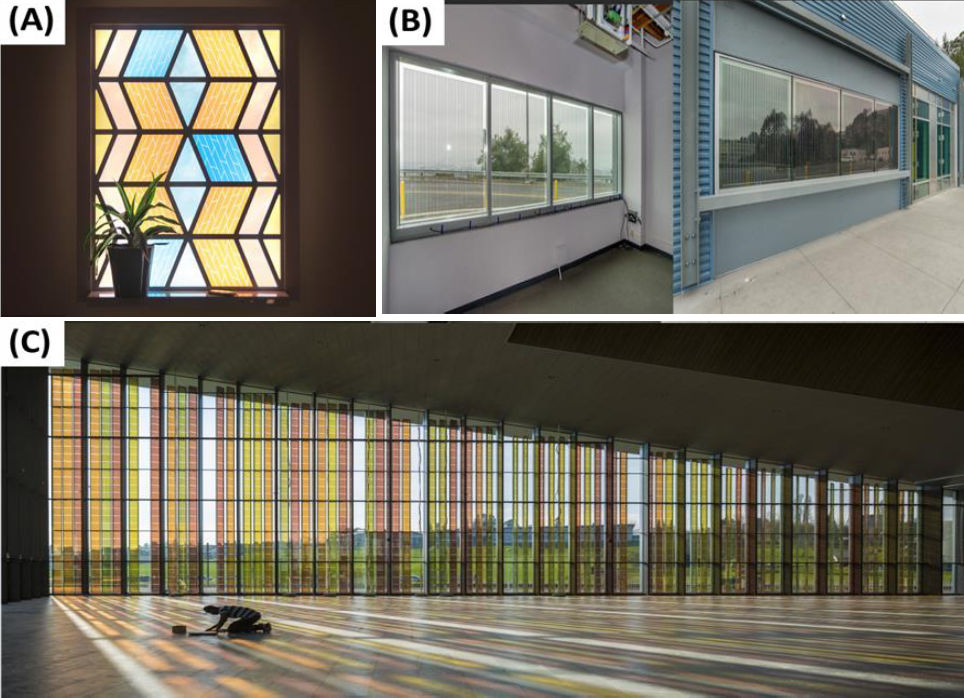
Figure 71. (A) Photovoltaic stained-glass window designed using dye synthesized solar cells utilizing different dye pigments to provide colour. (B) Semi-transparent organic PV window integrating a luminescent layer at Solaria, California. (C) Grätzel's Cell stained glass at the École Polytechnique Fédérale de Lausanne (CH).
Integration into the Building: System and Comfort
Similar to BIPV systems, continuous and low voltage is generated from the cells. To avoid DC-AC energy conversion losses from inverters, it is best to make direct use of the generated power in a local low power distribution network. To manage the operation and energy distribution, the system must be integrated with the building management system. A wide range of coloured devices can be offered thereby providing design aesthetics requirements while keeping the functionality of the underlying concept. Light absorption and rejection by the luminescent layer and semi-transparent photovoltaic cells limit solar transmission. The degree of light absorption can be modulated by the concentration of luminescent species and the cell transparency. Visible light (400nm – 800nm) transmission is typically around 10% to 50% with the transmission spectra controllable through the materials electro-optical properties (Ablayev et al. 2014; Romero-Gómez et al. 2015). There exists a trade-off between the cell efficiency and the transparency of the windows, in conjunction with location specific illumination conditions it may become customary to use numerical simulation and evaluation methodologies to evaluate the impact of each design choice (Saifullah, Gwak, and Yun 2016). As illustrated inFigure 71, the system is then a shading device simultaneously limiting glare and affecting occupant comfort and psychological response by the choice of colour rendered by the window.
SWOT Analysis
Strengths
- A renewable energy generation source is integrated to windows
- The ultraviolet part of the solar spectrum is converted to electrical power while further protecting the indoor environment from UV damages.
- It provides shading and glare protection in sunny climate with minimal or no visual obstruction through the window
- Design flexibility: the window opacity can be tuned on a case-by-case basis, there is no size or shape restriction and a choice of aesthetic colours is available
- The luminescent layers have a low cost and enhance the cell power conversion efficiency increase up to 3%
- The device is easy to install
- It is usable for many types of facades and roofs, without increasing the thickness of the structure
- There is potential for pre-fabrication
Weaknesses
- Matching the peak solar-to-electric power conversion efficiency of solar cells requires a coloured luminescent layer. Possible noncoloured layers would yield lower power conversion efficiency.
- There is a trade-off between power conversion efficiency and optical transparency
- Highly efficient luminescent species lack on the market as high performing materials are mostly confined to research environments
- There is no clear leading semi-transparent PV candidate (Organic, Perovskite, a-Si)
- There is lack of information regarding the photo-stability of luminescent candidates for the application, especially once embedded within a host material (polymer, glass etc.)
- Device designs may require their optical properties to be tailored towards a given buildings location (e.g. climate, distribution of structures in the surroundings)
Opportunity
- No LDS-PV commercial product is available yet, commercially available LSC based products start to appear
- The system application is supported by environmental policies and actions
- The integration with local DC networks is possible
- The systems can be integrated in new or refurbishing building designs
- It offers competitive advantages compared to fixed shading systems, coloured coatings, nonswitchable coloured glass
Threats
- The development of emerging electrically modulable switchable windows simultaneously providing power could overcome the performance of the system.
- The fluctuation of the electricity cost lead to uncertain payback time limiting the incentive on the technology integration
- Larger scale products and more business cases need to be developed for market persuasion and integration
Lessons Learned
- There is lack of an algorithm/software capable of evaluating the effect of each design consideration (building architecture and aesthetics, location, climate, PV technology, host material, luminescent species, optical transparency required etc.) on the buildings’ overall energy consumption.
- There is no clear definition of the optimal transparency across the visible region (400nm – 700nm) which bridges the gap between the semi-transparency and power conversion efficiency of the PV technology upon which a L-BIPV device is based.
- A critical evaluation of the luminescent material candidates is required under in-situ environmental conditions (temperature, spectral irradiance and relative humidity).
- Awaiting the design and discovery of luminescent structures capable of absorbing within the UV region, and re-radiating the energy close to the near infra-red where the majority of the PV technology currently has its peak responsivity.
- The window design must be flexible, allowing alterations for specific climate conditions i.e. more shade might be needed in warmer climates.
- There is lack of case-studies integrating the best performing materials (PV, host, and luminescent species) outlining the cost effectiveness of such structures in terms of their impact on building power management.
- A size limitation imposed by the optical properties of the individual design elements is yet to be defined allowing full scale structures to be fabricated and tested in different types of building architecture.
Further Reading
A. A. Hossam-Eldin, Mostafa Refaey, Abdelrahman Farghly. 2015. "A Review on Photovoltaic Solar Energy Technology and its Efficiency." In International Middle-East Power System Conference (MEPCON). Mansoura University, Egypt.
Ablayev, G. M., A. I. Kosarev, A. V. Kukin, M. Y. Semerukhin, M. Z. Shvarts, E. I. Terukov, and D. V. Zhilina. 2014. 'Semitransparent solar modules based on amorphous & microcrystalline silicon', Journal of Physics: Conference Series, 572: 012049. https://doi.org/10.1088/1742-6596/572/1/012049
Bouvard, Olivia, Sara Vanzo, and Andreas Schüler. 2015. 'Experimental Determination of Optical and Thermal Properties of Semi-transparent Photovoltaic Modules Based on Dye-sensitized Solar Cells', Energy Procedia, 78: 453-58. https://doi.org/10.1016/j.egypro.2015.11.696
Heim, Dariusz, Dominika Knera, and Anna Machniewicz. 2015. 'Modelling of Thermo-optical Properties of Amorphous and Microcrystalline Silicon Semitransparent PV Layer', Energy Procedia, 78: 430-34. https://doi.org/10.1016/j.egypro.2015.11.688
International-Renewable-Energy-Agency. 2012. "Solar Photovoltaics." In.
Klampaftis, Efthymios, David Ross, Keith R. McIntosh, and Bryce S. Richards. 2009. 'Enhancing the performance of solar cells via luminescent down-shifting of the incident spectrum: A review', Solar Energy Materials and Solar Cells, 93: 1182-94. https://doi.org/10.1016/j.solmat.2009.02.020
McKenna, Barry, and Rachel C. Evans. 2017. 'Towards Efficient Spectral Converters through Materials Design for Luminescent Solar Devices', Advanced Materials, 29: 1606491-n/a. https://doi.org/10.1002/adma.201606491
Romero-Gómez, Pablo, Francesco Pastorelli, Paola Mantilla-Pérez, Marina Mariano, Alberto Martínez-Otero, Xavier Elias, Rafael Betancur, and Jordi Martorell. 2015. "Semi-transparent polymer solar cells." In, 13. SPIE. https://doi.org/10.1117/1.JPE.5.057212